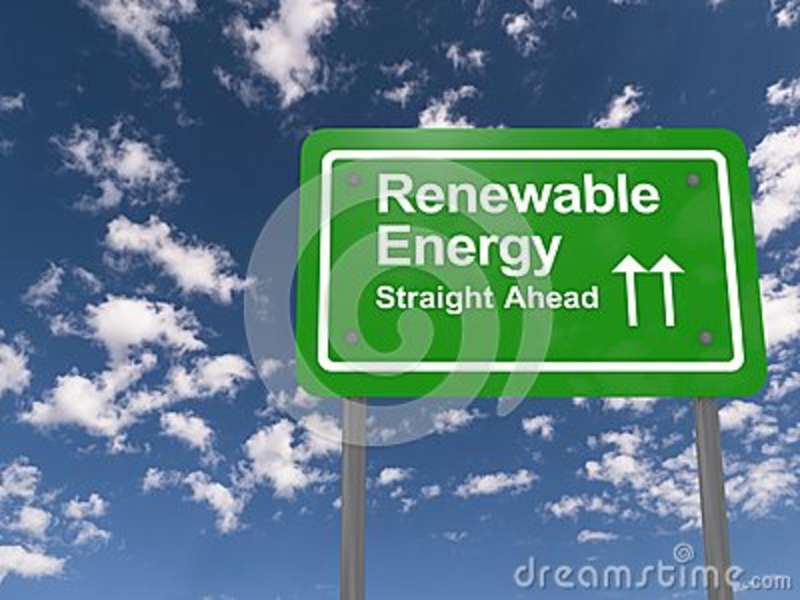
Renewable Energy Technologies
Renewable energy technologies produce sustainable, clean energy from sources such as the sun, the wind, plants, and water. According to the Energy Information Administration, in 2007, renewable sources of energy accounted for about 7% of total energy consumption and 9.4% of total electricity generation in the United States. Renewable energy technologies have the potential to strengthen our nation's energy security, improve environmental quality, and contribute to a strong energy economy.
Learn more about:
Biomass Technologies
There are many types of biomass—organic matter such as plants, residue from agriculture and forestry, and the organic component of municipal and industrial wastes—that can now be used to produce fuels, chemicals, and power. Wood has been used to provide heat for thousands of years. This flexibility has resulted in increased use of biomass technologies. According to the Energy Information Administration, 53% of all renewable energy consumed in the United States was biomass-based in 2007.
Biomass technologies break down organic matter to release stored energy from the sun. The process used depends on the type of biomass and its intended end-use.
Learn more about:
Geothermal Technologies
Geothermal technologies use the clean, sustainable heat from the Earth. Geothermal resources include the heat retained in shallow ground, hot water and rock found a few miles beneath the Earth's surface, and extremely high-temperature molten rock called magma located deep in the Earth.
Learn more about:
Hydrogen and Fuel Cell Technologies
Hydrogen is the simplest element on Earth. A hydrogen atom consists of only one proton and one electron. It is also the most plentiful element in the universe.
Despite its simplicity and abundance, hydrogen doesn't occur naturally as a gas on Earth. It is always combined with other elements. Water, for example, is a combination of hydrogen and oxygen. Hydrogen is also found in many organic compounds, notably the "hydrocarbons" that make up fuels such as gasoline, natural gas, methanol, and propane.
To generate electricity using hydrogen, pure hydrogen must first be extracted from a hydrogen-containing compound, then it can be used in a fuel cell.
Learn more about:
Hydropower Technologies
Hydropower, or hydroelectric power, is the most common and least expensive source of renewable electricity in the United States today. According to the Energy Information Administration, more than 6% of the country's electricity was produced from hydropower resources in 2008, and about 70% of all renewable electricity generated in the United States came from hydropower resources.
Hydropower technologies have a long history of use because of their many benefits, including high availability and lack of emissions.
Hydropower technologies use flowing water to create energy that can be captured and turned into electricity. Both large and small-scale power producers can use hydropower technologies to produce clean electricity.
Learn more about:
Ocean Energy Technologies
Oceans cover more than 70% of the Earth's surface. As the world's largest solar collectors, oceans contain thermal energy from the sun and produce mechanical energy from tides and waves. Even though the sun affects all ocean activity, the gravitational pull of the moon primarily drives tides, and wind powers ocean waves.
Learn more about:
Solar Energy Technologies
Solar energy technologies produce electricity from the energy of the sun. Small solar energy systems can provide electricity for homes, businesses, and remote power needs. Larger solar energy systems provide more electricity for contribution to the electric power system.
Learn more about:
Wind Energy Technologies
Wind energy technologies use the energy in wind for practical purposes such as generating electricity, charging batteries, pumping water, and grinding grain.
Most wind energy technologies can be used as stand-alone applications, connected to a utility power grid, or even combined with a photovoltaic system. For utility-scale sources of wind energy, a large number of turbines are usually built close together to form a wind farm that provides grid power. Several electricity providers use wind farms to supply power to their customers.
Stand-alone turbines are typically used for water pumping or communications. However, homeowners and farmers in windy areas can also use small wind systems to generate electricity. Learn more about small wind electric systems from Energy Saver.
Or learn more about:
Energy Management & Energy Efficiency
We perform energy and water audits for commercial & office buildings, schools, hotels, apartment properties, hospitals, and industrial / manufacturing facilities

"Demand Dollars fuel our New Economy”
Our Building Automation Industry can now demand dollars for giving back kW of electrical peak demand to the grid. This new reality is changing everything, while creating a new and very demanding Demand Response Industry. This new industry comes with new dollars for our industry which were previously spent on providing electrical generation and distribution.
Energy Efficiency Services
Whatever the size of your organisation, everyone stands to gain from being more energy efficient. Our range of services endeavour to identify, evaluate, and implement measures to save energy, with financial savings for the client being the priority.
Rising to the challenge – The whys and whens of renewable energy
If seems that no matter who you ask, renewable energy is finally considered a serious answer to .several of the world's most pressing problems - but why? In this report, we look at some of the reasons and discuss the economic feasibility of various electricity generation technologies as well as the implications of these findings for power system planners. Finally, they take a quick look at World Bank plans in this area.
The recent report by the Intergovernmental Panel on Climate Change (IPCC) should convince the few remaining skeptics that global warming is a fact, and this, coupled with the increasing convergence of the world's most influential policy makers that climate change must be dealt with as a top priority are sure to result in 1 mother shot in the arm for renewable energy technologies. With the world's energy needs increasing at a rapid rate, renewable energy and energy efficiency can help in ensuring these needs are met in an environmentally sustainable manner.
Even prior to the IPCC report, 2006 was a remarkable time for sustainable energy. Record amounts of venture capital flowed into the clean energy sector. Wall Street welcomed clean energy players with successful IPOs, and the popular press ran cover stories and special reports on carbon-free energy options, eco-friendly corporate practices and the greening of America.1Even Wal-Mart announced several initiatives embracing renewable energy and a powerful coalition of institutional investors continued to ask the world's largest public companies it information related to their greenhouse gas emissions under t Carbon Disclosure Project.3
After spending the last several decades in relative obscurity, is an opportune time for renewable energy practitioners to [themselves some questions. Why is renewable energy; an increasingly mainstream option? How prepared are we as practitioners to answer the barrage of feasibility-related questions that are bound to come our way? What is it that we still don't know?
WHY RENEWABLE ENERGY?
As mentioned, one of the main reasons why renewable energy is an increasingly attractive option is because it helps meet energy demand in an environmentally sustainable manner. But there are several other reasons which make renewable energy an increasingly feasible option.
Energy security
The recent volatility in the price of oil and natural gas has caused concern and disruption in both developing and developed countries. The electricity sector has been particularly affected as it remains overexposed to both oil and gas, In a World Bank supported research paper, Shimon Awerbuch and Martin Berger show that renewable energy systems broaden the portfolio of options for electricity resources.4 Renewable energy systems thus reduce the dependence on fuels with significant price volatility and availability concerns.
Awerbuch and Berger argue that the traditional least-cost approach of electricity planning is no longer suitable. According to them, electricity planners now have a wide range of technological and institutional options for generating electricity. Furthermore, the future that they are planning for is more dynamic, complex and uncertain. This makes predicting the long-run, least-cost alternative very difficult. They propose using a portfolio approach to value generating alternatives and energy diversity and security objectives. (The portfolio approach is a standard technique used by financial investors to diversify away idiosyncratic risk and increase expected returns.)
Using the portfolio approach, Awerbuch and Berger challenge the widely held belief that adopting renewable energy targets results in higher generation costs since renewable energy costs more on a stand-alone basis. They say that portfolio-based analyses in the US have indicated that adding PV wind and other fixed-cost renewable technologies to a fossil generating portfolio lowered the overall generating cost and risk. They explain this as resulting from the portfolio effect.
They then went on and evaluated the EU renewables targets and energy security objectives using a similar approach and discovered that the EU generating mixes are sub-optimal because it is possible to have portfolios with lower costs and risk by including more wind or other fixed-cost renewables. Not only does this reduce energy cost but it also increases energy security. They also show how introducing renewables like wind and geothermal technologies into the electricity generating mix for Mexico can reduce both the cost and the risk of future electricity generation. Thus, there is a possibility that all electricity generating portfolios can reduce costs and risks by including some portion of fixed-cost renewable generation.
Economic valuation
Traditional financial analysis is based on discounted cash-flow accounting. This sort of analysis fails to adequately capture the future fuel price risks. It also completely ignores the environmental and health costs of fossil-fuelled power plant emissions. Consequently, most countries utilize modern renewable energy (excluding traditional biomass) in an extremely limited way. Yet, if we consider the life-cycle cost, some renewable energy technologies are already cost competitive -with conventional energy sources. Despite this, the potential of these financially viable renewable energy technologies is not fully realized because of a variety of market barriers, such as large public subsidies for conventional fuels (Figure 1), lack of adequate regulatory and legal frameworks in support of renewable energy, and undeserved risk premiums built into renewable energy deals, making access to credit difficult.5
If we include the costs of local environmental externalities and global externalities (e.g. through carbon credits) in the economic valuation, as is done in a typical World Bank economic calculation (when projects could benefit form carbon financing), then the economic viability of renewable energy increases. Furthermore, if the diversification value (as discussed above) is also added to the economic cost, then the economically viable optimum quantity of renewable energy increases even more. Such economic analyses have been performed for various renewable energy projects, for example in China, Croatia, Mexico and South Africa, to determine the economically viable optimum quantity of renewable energy and these can be found on the World Bank's energy website.6
Achieving the MDGs
For those of us in the international development sector, the Millennium Development Goals (MDGs) are the 'Holy Grail'. For a renewable energy practitioner, it is important to know that the international development agenda is driven by the MDGs. The MDGs are eight goals (see boxed text) which the world community (countries, multilateral banks, development agencies, NGOs, United Nations) has set for itself in order to mount a focused fight against poverty. The MDGs grew out of the agreements and resolutions of world conferences organized by the United Nations in the past decade. In September 2000, the 189 member states of the UN unanimously adopted the Millennium Declaration, which outlined these goals. The first seven goals focus the world community's attention on particular aspects of poverty. The eighth goal focuses on an ideal macroeconomic environment which would facilitate the achievement of the first seven goals. Each of the first seven MDGs has definite quantifiable targets (total 15) and is also time bound. Thus, the MDGs create a sense of urgency. They also come with indicators (48 in total) to monitor progress. Most of the MDGs are supposed to be achieved by 2015.
Coal
Although the MDGs do not specifically mention energy, energy plays an essential role in their achievement. According to the International Energy Agency estimates, in order to meet the MDG poverty-reduction target, modern energy services will have to be provided to an additional 700 million people by 2015.Two very interesting papers highlight the role that energy and renewable energy have to play in achieving the MDGs. The first paper, by the UN, called The Energy Challenge for Achieving the Millennium Development Goals discusses the direct and indirect role that energy can play in achieving each of the eight MDGs.7 For example; the second MDG is to achieve universal primary education. Energy can help achieve this goal by providing after-dusk illumination which will enable students to study. Electrified rural schools and villages also make it easier to attract teachers to rural areas.
The second paper - by The Worldwatch Institute - is called Energy for Development: The Potential Role of Renewable Energy in Meeting the Millennium Development Goals.8 This paper says that renewable energy can contribute both directly and indirectly. It contributes directly to poverty alleviation by providing the energy needed for creating businesses and jobs. This turns locally available resources into productive economic assets. The paper also provides several examples of the indirect ways in which renewable energy can help facilitate achieving the MDGs. For example, locally produced biomass reduces expenses and frees up time for learning and income-generating opportunities. Renewable energy for cooking and heating reduces the time that children, especially girls, spend out of school collecting fuel. Renewable energy also contributes to improved health by providing energy to refrigerate medicine, sterilize medical equipment and incinerate medical waste. Thus, the MDGs are also playing a part in creating a more mainstream role for renewable energy.
Assessment of various electricity generation technologies
As the excitement over the potential of renewable energy continues to grow, it makes obvious the need for more knowledge. It is essential that both renewable energy practitioners as well as energy planners have a good sense of just exactly when renewable energy is feasible and when is it not. The China study mentioned above underscored the importance of having robust and credible knowledge.
So far we have been having this discussion using the umbrella term 'renewable energy', but, in reality, various renewable energy technologies are as different from one another as nuclear energy is from coal-fired generation. Thus, it is important to get a good sense of which renewable energy technologies are competitive and which are not. Also important to consider are the various configurations: off-grid, mini-grid and grid. Furthermore, any feasibility analysis is extremely sensitive to the time period under consideration, and thus a robust analysis must consider various time period scenarios as well. Anticipating the need for a sophisticated and exhaustive analysis "which takes all of the above-mentioned factors into account, the World Bank commissioned a study. This electrification assessment study was undertaken by a team comprising Chubu Electric Power Co Inc, Toyo Engineering Corporation, Princeton Energy Resources International, Energy Technologies Enterprises Corp, Global Energy Associates Inc, and The Energy and Resources Institute.
Scope of the study
The study examined power generation technologies across a size range of 50 W to 500 MW organized into three distinct electricity delivery configurations: off-grid, mini-grid and grid (see Table 1). Generation technologies examined included renewable energy technologies (photovoltaic’s, wind, geothermal, hydro, biomass-electric, biogas-electric); conventional generation technologies (gasoline or diesel generator; oil/gas steam-electric, combustion turbines and combined cycle; coal-fired steam-electric, oil/gas-fired combustion turbine and combined cycle technologies, and coal-fired and oil-fired steam cycle technologies); and emerging technologies (integrated gasification combined cycle, atmospheric fluidized bed combustion, fuel cells and micro-turbines).The economic assessment was performed for
It is essential that both renewable energy
practitioners as well as energy planners have
a of just exactly when renewable
Energy is feasible
Three different time periods (2005, 2010 and 2015) in order to incorporate projected cost reductions from scaling-up of emerging technologies. A levelized analysis of capital and generation costs was conducted in economic, rather than financial, terms to allow generic applications of results to any developing country. Capital and generation cost projections
Incorporated uncertainty analysis, allowing the results to reflect sensitivity to key input assumptions. The study results make it possible to compare the levelized economic costs of electricity technologies over a broad range of deployment modes and demand levels, both at present and in future.
Methodology of the study
A five-step process comprised the methodology. First, a technology assessment was performed for each candidate generation technology. The assessment covered operating principles, application for electrification purposes, and prospects for performance improvement and capital cost reduction. Next, they did an environmental characterization which focused on typical environmental impacts from normal operations using typical emissions control measures and costs. The assessment assumed use of emissions controls in accordance with World Bank environmental guidelines; these costs were included in the economic assessment. The third step was to do a capital cost assessment using a standard mathematical model and actual cost data (where available) and reflecting typical deployment. Future capital costs of generation were then developed, based on technology forecasts (e.g. learning curves) and incorporating uncertainties in equipment cost, fuel cost and capacity factor. The uncertainty analysis was a parametric analysis of variability in key inputs and generated a band of maximum and minimum costs for each period (2OO5, 2O10 and 2O15). Finally, levelized generating costs were calculated using a consistent economic analysis method but differentiated according to deployment conditions. This last step also included an uncertainty analysis on the inputs to the levelized cost calculation, again generating a band of maximum and minimum costs for the 2005, 2010 and 2015 periods. All cost estimates were developed for a single reference location (India) to minimize any site-specific discrepancies when comparing technologies.
Key findings
The study revealed emerging trends in terms o the relative economics of renewable and conventional generation technologies? According to size and configuration. In interpreting and applying these findings it should be kept in mind that the assessment effort is a desk study bound by time (technology and prices are not static) and method (it consolidates secondary source information rather than generating new content).
Renewable energy is more economical than conventional generation for off-grid (less than 5 kVU) applications.
Several renewable energy technologies -wind, mini-hydro and biomass-electric - can deliver the lowest levelized generation costs for off-grid electrification (see Figure 2), assuming availability of the renewable resource. Pico-hydro in particular can deliver electricity for 10-20 cents/kWh, less than one-quarter of the 40-60 cents/kWh for comparably-sized gasoline and diesel engine generators. Even relatively expensive renewable energy technology (solar PV) is comparable in levelized electricity costs to the small fuel-using engine-generators under 1 kW in size.
Several renewable energy technologies are potentially the least-cost, mini-grid generation technology.
Mini-grid applications are village and district-level isolated networks with loads of 5 kW -500 kW. The assessment results suggest several renewable energy technologies (biomass, geothermal, wind and hydro) may be the most economical generation choice for mini-grids, assuming a sufficient renewable resource is available (see Figure 3). Two biomass technologies - biogas digesters and biomass gasifiers - seem particularly promising due to their high capacity factors and availability in size ranges matched to mini-grid loads. Since so many renewable energy sources are viable in this size range, mini-grid planners should thoroughly review their options to make the best selection.
Conventional power generation technologies
For the foreseeable future, conventional power generation technologies (open cycle and combined cycle gas turbines, coal- and oil-fired steam turbines) remain more economical for most large grid-connected applications, even with increases in oil price forecasts (see Figure 4). Site-specific considerations such as load profile demand and cost differentials between oil, natural gas and coal prices determine which configuration is the least expensive. Using super-critical or ultra-supercritical (USC) for very large (over 500 MW) power plants is most cost-effective when fuel prices are high and CO2 reductions are sought.
Two new coal technologies have considerable potential for developing economics
Two new coal-fired power plant technologies - IGCC and AFBC - are attracting considerable attention by planners of large power grids in countries with coal or lignite reserves. [4FBC is already commercially available up to 300 MW size and used widely worldwide, including in China and India. This technology is competitive in situations where low-quality inexpensive fuel is available and when SO2 emission regulations a wet scrubber. In the 100 MW to 300 MW range, the circulating fluidized bed (CFB) option is preferable. The AFBC option may also be applicable to smaller thermal power plants (under 100 MW) using biomass and municipal solid wastes (MSW). IGCC is in the early commercialization stage and could become a viable and competitive option in the future given its excellent environmental performance.
Implications for power system planners
Power system planners tend to operate on an incremental basis, with new capacity additions selected to accommodate the location and pace of load growth on a least-cost basis. The study suggests that scale is a critical aspect affecting the economics of different generation configurations. When a national or regional grid is developed with sufficient transmission capacity, and incremental load growth is fast, large, central-station gas combined cycle and coal fired power plants would clearly be the least-cost alternatives. However, based on site-specific characteristics, if the size of the grid is limited or the incremental load growth is small, it may make economic sense, reduce financing and construction risk and secure power system stability to add several smaller power stations rather than one very large power station.
Furthermore, if local resources such as indigenous coal, gas, biomass or geothermal or wind or hydro are used, this may increase energy security and mitigate some of the uncertainty caused by volatile international fuel prices.
More research required
The findings reached by the study suggest that choosing generation technologies and electrification arrangements is becoming a more complicated process. New technologies are becoming more economical and technologically mature, uncertainty in fuel and other inputs is creating increasing risk regarding future electricity costs, and old assumptions about economies of scale in generation may no longer be valid. Although the assessment methods used in the study provided a useful comparison among technologies, there needs to be further refinement in the methodology. That will generate even more confidence in the conclusions reached, which can then become the basis of national or regional electrification plans.
Accounting for the locational and stochastic variability of renewable resources, as well as balancing costs, land costs, labor and transport costs, all need further investigation, as does the method of accounting for the incremental cost of delivering electricity. The need to accommodate environmental externalities in the economic assessment also needs more attention. Finally, the relative economics of conventional vs. renewable energy is largely driven by forecasts of fuel prices together with certain construction and manufacturing materials prices, such as steel, concrete, glass and silicon. All these commodity prices are increasingly subject to uncertainties and price fluctuations in possibly countervailing directions, which make forecasts of future generation costs extremely uncertain. Since the study was conducted, there have been cost increases in coal-fired power plants, gas and steam turbine prices, wind energy and, photovoltaic modules. This will have an impact on the findings of the study.
Cost estimates for electricity from US coal fired power plants used to hover around the US$1000 per kW mark but were closer to $1400 per kW in 2006. Gas and steam turbine!
Prices have seen more than a 50% increase because of strong demand from oil producing countries. Wind farm bids are coming in at $1700-$2000 compared to much more modest
assumptions in study. Recent (2007) bids for World Bank and GEF-financed solar thermal power plants have been about 1.5 times more than estimated costs. Similarly, for photovoltaic modules the study assumes continued decreases in costs off 20% between 2004 and 2015 due to technology advancement and growing production volume; whereas costs have actually been climbing steadily, as shown in Figure 5.
In the light of the above, it is obvious that additional work, including use of hedging or other financial risk mitigation instruments, is needed to quantify and reflect these future fuel and commodity price uncertainties as part of the electrification planning process. Following in the footsteps of Awerbuch and others, the World Bank has begun to investigate alternative electric power systems planning models that explicitly incorporate risks and uncertainties in the decision process. A workshop to examine the issues and to develop a plan of action was hosted recently at the World Bank.9
Role of the World Bank
The World Bank Group (WBG) is committed to promote not just investments but also adequate policy environments supporting renewable energy. In September 2005, after the Gleneagles G8 summit, the WBG began preparing a Clean Energy and Development Investment Framework. The investment framework covers three inter-related areas.
Pillar 1 focuses on the role of energy for economic growth and development. It includes issues such as access for the poor and poverty reduction. Pillar 2 focuses on the policies and financial requirements to support a transition to a low-
The World Bank Group is committed to
Promote not just investments but also
Adequate policy environments supporting renewable energy
Carbon economy. Pillar 3 focuses on the need for investments to reduce vulnerability to climate variability, especially for the poor, who suffer the most from this problem. The investment framework is intended to be a vehicle to accelerate investment along each of these three pillars. Renewable energy will be a key ingredient as the WBG moves forward on Pillars 1 and 2.
The WBG has begun implementing the Investment Framework for Clean Energy for Development.10 this offers a structure upon which the global community can direct its support for renewable energy in the coming years. Although this article is focused on renewable energy, from a climate change standpoint we cannot ignore the vast untapped potential of the 'cheapest' source of energy - energy efficiency. Renewable energy and energy efficiency development must go hand in hand.
The growth of the WBG RE and EE portfolio is evidence of this. Since 1990, the WBG has committed more than $10 billion to renewable energy and energy efficiency. Since the Bonn Renewable Energy Conference in 2004, our new renewable energy and energy efficiency commitments have accelerated, reaching $1.13 billion in the past two fiscal years. In fiscal 2006, commitments for new renewable energy and energy efficiency were $668 million, growing at more than double the 20% Bonn growth target.11
Although a lot has been accomplished by the WBG in this area, it is extremely aware that to fully integrate RE and EE into global markets, there are several issues that will need to be addressed. Some of these issues are:
• Increased access to long-term financing to make capital intensive renewable energy technologies financially viable and
Affordable.
• Increased access to long-term financing to make capital intensive renewable energy technologies financially viable and
Affordable.
• More effective technology transfer and strengthening the capacity to plan, supply, install and maintain technologies
• Making energy prices reflect full costs of supply and eliminating fuel subsidies
• Reducing perceived risk and high cost of RE/EE transactions
• Improving regulatory and policy
Frame works and establishing the enabling legislation
Moving forward, the WBG's Investment Framework will serve as a platform for assisting partner countries to meet energy demand and incorporate low-carbon technologies such as renewable energy and energy efficiency into their investment strategies.
REFERENCES
1. Please see the September 2006 Special Issue of Scientific American; the Special Report in the 29 January 2007 issue of Business Week, the Special Report in the 27 January 2007 issue of The Economist, cover story called
'Green is Good' in Information Week (March 12,2007); cover story called 'Green dreams - The risky boom in the clean-energy business' in The Economist's November 2006); cover story called 'Go Green. Get Rich.' in Business 2.0 (January/February 2007); and the Energy Journal Report in The Wall Street Journal (February 12, 2007)
2. See Wal-Mart website at
www.walmartstores.com/GlobalWMStoresWeb/navigate.do?catg=678
3. See Carbon Disclosure Project at www.cdproject.net
4. S. Awerbuch, and M. Berger, 'A Portfolio Approach to Energy Planning n Mexico,' The World Bank, Washington DC. Report available at www.worldbank.org/retoolkit
5. International Energy Agency, 'World Energy Outlook 2006,' Paris, France
6. Reports available at www.worldbank.org/retoolkit
7. UN Energy, The Energy Challenge for Achieving the Millennium Development Goals,' United Nations, New York, NY. http://esa.un.org/un-energy/pdf/UN-ENRG%20paper.pdf
8. The Worldwatch Institute, 'Energy for Development: The Potential Role of Renewable Energy in Meeting the Millennium Development Goals, Renewable Energy Network for the 21st Century, Paris, France. www.ren21 .net
9. Donald Hertzmark, 'Risk Assessment Methods for Power Utility Planning,' Energy Sector Management Assistance Programme Special Report 001/07, The World Bank, Washington DC, March 2007. www.worldbank.org/re
10. The World Bank, 'An Investment Framework for Clean Energy for Development,' Washington DC. See http://www.worldbank.org/sustainabledevelopment
11. The World Bank, 'Improving Lives: World Bank Group Progress on Renewable Energy & Energy Efficiency in Fiscal Year 2006,' Energy and Mining Sector Board, Washington DC, December 2006. http://www.worldbank.org/re
Millennium Goals
7. Ensure environmental sustainability: Energy production, distribution and consumption has many adverse effects on the local, regional and global environment, including indoor, local and regional air pollution, local particulates, land degradation, acidification of land and water, and climate change. Cleaner energy systems are needed to address all of these effects and to contribute to environmental sustainability.
8. Develop a global partnership for development: The World Summit for Sustainable Development called for partnerships between public entities, development agencies, civil society and the private sector to support sustainable development, including the delivery of affordable, reliable and environmentally sustainable energy services.
Inverter and charge controllers are supplied in 12v/24v or 48v dependent on the voltage of the ULTRA X900 required.
|

ULTRA X900 System details
Number of blades: 3
Blade material: Carbon fiber
Rotor Diameter(m): 2.3
Swept area 4.15 m2
Mast head weight:21.3kg
Start up wind speed: 2.7ms
Generator: Permanent magnetic 3 phase
Max.output power(W): 1500
Output voltage(VDC): 12, 24 or 48
Wind direction: Tail rudder
Speed limiter: Automatic
3 stage Auto Furl overspeed protection
Tower fixings: Wire guy
Altitude of tower: 6mt to 24mt
Charging control: Automatic
Sine wave inverter: 12, 24 or 48v input
Guarantee: 2 years
Options
Battery packs: 1,2 or 4 x 12v/200ah
SMA grid connection inverter
Marine grade stainless steel tower kit
Free standing tower kits
Hybrid PV system design
|
Brochure click here |
|
UNIQUE FURLING SYSTEM
|
With a smaller diameter, lighter weight and very stiff blade technology the ULTRA X900 out performs higher rated competitors in medium to high wind conditions. That's because ULTRA does not ‘hide’ from the wind but maintains its full performance throughout its entire flight. Most small wind turbines tend to turn off or turn away from the wind as wind speed increases, this reduces their output.
The ULTRA X900 range employs a unique, 3 stage, upward furling design so that production continues even in strong winds. Above 30mph the ULTRA continues to produce peak power in excess of 1500W and some users report up to 1800W at 100amps without damage to either electrical or mechanical components. Rain, snow, sleet or storms this wind turbine is made to take it all and continue to provide optimum power. |
The turbine uses an 8 pole, 36 slot, 3 phase alternator with 10 winding leads. This allows 4 separate field adjustable settings to be made that will optimise it's output and match it's performance to low, medium, high or severe wind regimes.
ULTRA turbines are sealed units and require only annual inspection. No lubrication or overhaul maintenance schedule is required during the life of the generator. (approx. 15-20 years). Marine or desert installations may need more attention to surface corrosion and leading-edge tape on the blades. Helicopter grade leading-edge tape is replaceable if abraded by fine debris or sand.
|
 |
ULTRA field units have survived 120mph storms while other units nearby have self-destructed. Vehicle mounted test units continue to output maximum power at over 70mph with no damaging effects. ULTRA aerospace grade uni-directional carbon fiber blades are not to be confused with carbon matrix or carbon reinforced plastic blades. Unlike plastic with powdered carbon blades that bend and flutter, the carbon fibers in the ULTRA blades are 7 times stiffer than common steel, and carry the loads from tip to root. This allows the turbine to harness far more energy annually than comparable similar wind turbine generators. In addition, the increased output substantially lowers the overall cost of energy despite a slightly higher initial purchase price.
The ULTRA 900W has a special UV stabilized titanium dioxide, linear polyurethane aircraft coating that protect the unit from rain, snow, ice, sun, sand and dust, wind and corrosion. In addition, since the blades are very light they can take advantage of wind gusts that heavier blades can't because their inertia is too high to react before the gust is over. Over time this amounts to considerable extra energy extracted from the same column of air.
|
Most Americans support an increasing role for renewable energy. Renewable energy comes from local resources, so it improves national security; creates jobs in rural America and in manufacturing; provides energy at stable prices that will never run out; and reduces urban smog and pollution that contributes to global warming.
Renewable energy can be harnessed in every one of our 50 states-from resources like wind, solar, hydro, and biofuels. 20 states and the District of Columbia already require a minimum level of renewable energy in their electric power consumption. Renewable energy, in the form of biofuels, can also be used in the transportation sector to power our cars and trucks, which reduces our dependence on oil from the Middle East.
To learn more about renewable energy follow these links:
Renewable Fuels: Biofuels >
Solar >
Wind >
Hydropower >
Geothermal >
Energy Efficiency >
|
|
Wind Energy Systems
|
|
|
Main Components of a Wind Turbine

Rotor
The portion of the wind turbine that collects energy from the wind is called the rotor. The rotor usually consists of two or more wooden, fiberglass or metal blades which rotate about an axis (horizontal or vertical) at a rate determined by the wind speed and the shape of the blades. The blades are attached to the hub, which in turn is attached to the main shaft.
Drag Design
Blade designs operate on either the principle of drag or lift. For the drag design, the wind literally pushes the blades out of the way. Drag powered wind turbines are characterized by slower rotational speeds and high torque capabilities. They are useful for the pumping, sawing or grinding work that Dutch, farm and similar "work-horse" windmills perform. For example, a farm-type windmill must develop high torque at start-up in order to pump, or lift, water from a deep well.
Lift Design
The lift blade design employs the same principle that enables airplanes, kites and birds to fly. The blade is essentially an airfoil, or wing. When air flows past the blade, a wind speed and pressure differential is created between the upper and lower blade surfaces. The pressure at the lower surface is greater and thus acts to "lift" the blade. When blades are attached to a central axis, like a wind turbine rotor, the lift is translated into rotational motion. Lift-powered wind turbines have much higher rotational speeds than drag types and therefore well suited for electricity generation.
Tip Speed Ratio
The tip-speed is the ratio of the rotational speed of the blade to the wind speed. The larger this ratio, the faster the rotation of the wind turbine rotor at a given wind speed. Electricity generation requires high rotational speeds. Lift-type wind turbines have maximum tip-speed ratios of around 10, while drag-type ratios are approximately 1. Given the high rotational speed requirements of electrical generators, it is clear that the lift-type wind turbine is most practical for this application.
The number of blades that make up a rotor and the total area they cover affect wind turbine performance. For a lift-type rotor to function effectively, the wind must flow smoothly over the blades. To avoid turbulence, spacing between blades should be great enough so that one blade will not encounter the disturbed, weaker air flow caused by the blade which passed before it. It is because of this requirement that most wind turbines have only two or three blades on their rotors.
Generators
The generator is what converts the turning motion of a wind turbine's blades into electricity. Inside this component, coils of wire are rotated in a magnetic field to produce electricity. Different generator designs produce either alternating current (AC) or direct current (DC), and they are available in a large range of output power ratings. The generator's rating, or size, is dependent on the length of the wind turbine's blades because more energy is captured by longer blades.
It is important to select the right type of generator to match your intended use. Most home and office appliances operate on 120 volt (or 240 volt), 60 cycle AC. Some appliances can operate on either AC or DC, such as light bulbs and resistance heaters, and many others can be adapted to run on DC. Storage systems using batteries store DC and usually are configured at voltages of between 12 volts and 120 volts.
Generators that produce AC are generally equipped with features to produce the correct voltage (120 or 240 V) and constant frequency (60 cycles) of electricity, even when the wind speed is fluctuating.
DC generators are normally used in battery charging applications and for operating DC appliances and machinery. They also can be used to produce AC electricity with the use of an inverter, which converts DC to AC.
Transmission
The number of revolutions per minute (rpm) of a wind turbine rotor can range between 40 rpm and 400 rpm, depending on the model and the wind speed. Generators typically require rpm's of 1,200 to 1,800. As a result, most wind turbines require a gear-box transmission to increase the rotation of the generator to the speeds necessary for efficient electricity production. Some DC-type wind turbines do not use transmissions. Instead, they have a direct link between the rotor and generator. These are known as direct drive systems. Without a transmission, wind turbine complexity and maintenance requirements are reduced, but a much larger generator is required to deliver the same power output as the AC-type wind turbines.
Towers
The tower on which a wind turbine is mounted is not just a support structure. It also raises the wind turbine so that its blades safely clear the ground and so it can reach the stronger winds at higher elevations. Maximum tower height is optional in most cases, except where zoning restrictions apply. The decision of what height tower to use will be based on the cost of taller towers versus the value of the increase in energy production resulting from their use. Studies have shown that the added cost of increasing tower height is often justified by the added power generated from the stronger winds. Larger wind turbines are usually mounted on towers ranging from 40 to 70 meters tall.
Towers for small wind systems are generally "guyed" designs. This means that there are guy wires anchored to the ground on three or four sides of the tower to hold it erect. These towers cost less than freestanding towers, but require more land area to anchor the guy wires. Some of these guyed towers are erected by tilting them up. This operation can be quickly accomplished using only a winch, with the turbine already mounted to the tower top. This simplifies not only installation, but maintenance as well. Towers can be constructed of a simple tube, a wooden pole or a lattice of tubes, rods, and angle iron. Large wind turbines may be mounted on lattice towers, tube towers or guyed tilt-up towers.
Installers can recommend the best type of tower for your wind turbine. It must be strong enough to support the wind turbine and to sustain vibration, wind loading and the overall weather elements for the lifetime of the wind turbine. Tower costs will vary widely as a function of design and height. Some wind turbines are sold complete with tower. More frequently, however, towers are sold separately.
Basic Designs
Wind turbines are classified into two general types: horizontal axis and vertical axis. A horizontal axis machine has its blades rotating on an axis parallel to the ground. A vertical axis machine has its blades rotating on an axis perpendicular to the ground. There are a number of available designs for both and each type has certain advantages and disadvantages. However, compared with the horizontal axis type, very few vertical axis machines are available commercially.
Horizontal Axis
This is the most common wind turbine design. In addition to being parallel to the ground, the axis of blade rotation is parallel to the wind flow. Some machines are designed to operate in an upwind mode, with the blades upwind of the tower. In this case, a tail vane is usually used to keep the blades facing into the wind. Other designs operate in a downwind mode so that the wind passes the tower before striking the blades. Without a tail vane, the machine rotor naturally tracks the wind in a downwind mode. Some very large wind turbines use a motor-driven mechanism that turns the machine in response to a wind direction sensor mounted on the tower.
Vertical Axis
Although vertical axis wind turbines have existed for centuries, they are not as common as their horizontal counterparts. The main reason for this is that they do not take advantage of the higher wind speeds at higher elevations above the ground as well as horizontal axis turbines. The basic vertical axis designs are the Darrieus, which has curved blades, the Giromill, which has straight blades, and the Savonius, which uses scoops to catch the wind.
A vertical axis machine need not be oriented with respect to wind direction. Because the shaft is vertical, the transmission and generator can be mounted at ground level allowing easier servicing and a lighter weight, lower cost tower. Although vertical axis wind turbines have these advantages, their designs are not as efficient at collecting energy from the wind as are the horizontal machine designs.
Augmentors
Some experimental wind turbines have incorporated an added structural design feature, called an augmentor, intended to increase the amount of wind passing through the blades. However, these augmentors do not increase the energy capture of the machine enough to justify the added cost of employing them.
Operating Characteristics
All wind machines share certain operating characteristics, such as cut-in, rated and cut-out wind speeds.
Cut-in Speed
Cut-in speed is the minimum wind speed at which the wind turbine will generate usable power. This wind speed is typically between 7 and 10 mph.
Rated Speed
The rated speed is the minimum wind speed at which the wind turbine will generate its designated rated power. For example, a "10 kilowatt" wind turbine may not generate 10 kilowatts until wind speeds reach 25 mph. Rated speed for most machines is in the range of 25 to 35 mph. At wind speeds between cut-in and rated, the power output from a wind turbine increases as the wind increases. The output of most machines levels off above the rated speed. Most manufacturers provide graphs, called "power curves," showing how their wind turbine output varies with wind speed.
Cut-out Speed
At very high wind speeds, typically between 45 and 80 mph, most wind turbines cease power generation and shut down. The wind speed at which shut down occurs is called the cut-out speed. Having a cut-out speed is a safety feature which protects the wind turbine from damage. Shut down may occur in one of several ways. In some machines an automatic brake is activated by a wind speed sensor. Some machines twist or "pitch" the blades to spill the wind. Still others use "spoilers," drag flaps mounted on the blades or the hub which are automatically activated by high rotor rpm's, or mechanically activated by a spring loaded device which turns the machine sideways to the wind stream. Normal wind turbine operation usually resumes when the wind drops back to a safe level.
Betz Limit
It is the flow of air over the blades and through the rotor area that makes a wind turbine function. The wind turbine extracts energy by slowing the wind down. The theoretical maximum amount of energy in the wind that can be collected by a wind turbine's rotor is approximately 59%. This value is known as the Betz limit. If the blades were 100% efficient, a wind turbine would not work because the air, having given up all its energy, would entirely stop. In practice, the collection efficiency of a rotor is not as high as 59%. A more typical efficiency is 35% to 45%. A complete wind energy system, including rotor, transmission, generator, storage and other devices, which all have less than perfect efficiencies, will (depending on the model) deliver between 10% and 30% of the original energy available in the wind.
Sizing Grid Connected Systems
The size, or generating capacity, of a wind turbine for a particular installation depends on the amount of power needed and on the wind conditions at the site. It is unrealistic to assume that all your energy needs can be met economically by wind energy alone. As a general rule, a wind system should be sized to supply 25% to 75% off your energy requirements. Most residential applications require a machine capacity of between 1 and 10 kW. Farm use requires 10 to 50 kW and commercial/small industrial uses typically require 20 kW or larger.
Because most buildings are connected to a utility line, many wind turbine owners have opted to interconnect their systems. In effect, they use the utility as a backup system. Excess electricity from the turbine is automatically fed to the utility and backup power is automatically supplied. While this does not constitute true storage, it provides power on demand at any time, in any amount. The process to obtain approval for interconnection from the utility company can be lengthy and complicated, and requires careful planning. The possibilities for interconnection should be investigated early in the process of researching a wind system.
The variability of your energy consumption and the amount of money you are willing to spend on a wind system should also guide your selection. For example, a user whose consumption is erratic or concentrated during short periods of the day should size a wind turbine differently than a user with a fairly constant energy demand. In the former case, wind turbine size should be a function of off-peak or average energy demand.
Off-Grid Systems
Off-grid power systems can result in higher cost energy, but the high cost of extending a power line to a remote location often makes an independent energy system the most cost effective choice for remote homes and equipment. If the average wind speeds at a location are greater than 12 mph, a wind turbine may provide the least expensive form of energy.
Because wind is intermittent, it is often used in conjunction with batteries or with other energy sources, such as a gas generator or solar electric panels, to make a hybrid system. Battery systems can supply the owner with reserve power whenever energy demand exceeds that delivered by the wind turbine. This reserve power comes in handy during calm spells, but in situations where the storage capacity is taxed beyond its limits, a backup system, such as a portable gasoline or diesel generator, may be necessary. By combining two or more sources of energy, the size of energy storage can be decreased.
Energy Storage
Electrical Storage
Batteries are the most common form of electrical storage. Where heat, rather than electricity, is the desired end product of a wind turbine application, hot water is the usual storage medium. However, the advantageous economics of other heating systems make wind-powered heating a less attractive option.
Batteries can store and deliver only DC power. Unless an inverter is used to convert DC to AC, only DC appliances can be operated from the stored power. The battery voltage must be the same as the voltage needed to run the appliance. Standard battery voltage is 6 or 12 volts. For an appliance requiring 24 volts, two 12-volt or four 6-volt batteries connected in series are required. For a 120-volt application, you will need a series of ten 12-volt batteries.
The least costly batteries for wind applications are deep cycle, heavy-duty, industrial type lead-acid batteries, such as those used in golf carts and forklifts designed for high reliability and long life. They can be fully charged and discharged, while standard lead-acid batteries (e.g., automobile type) cannot. Gel-cell lead acid batteries have improved the safety of the traditional liquid acid battery by containing the hydrogen that can be produced during charging, and by preventing the liquid acid from spilling.
Battery conversion efficiency is approximately 60% to 80%. A battery's capacity is rated in amp-hours, a measure of its ability to deliver a certain amperage for a certain number of hours. For example, for a rating of 60 amp-hours, 3 amps can be delivered for 20 hours. Batteries should be routinely inspected for fluid level and corrosion. The storage room should be well ventilated. If allowed to accumulate, the hydrogen gas produced by some batteries can explode.
Substantial research on battery technology has taken place since 1990, when the Federal Clear Air Act Amendment prompted new battery research for electric vehicles. Much of this research has focused on developing batteries that can be rapidly charged and are lighter in weight. Many new types of batteries are being developed. Two examples of near-future alternatives to lead-acid batteries are nickel-cadmium batteries and nickel-iron batteries. Both types generally provide good low-temperature performance and long life, but they are still more expensive than lead-acid batteries.
Heat Storage
When heat is the desired end product, hot water is an alternate way to store energy. It is well suited to northern climates where the heating season coincides with the windy season. There are two basic ways to produce heat from a wind turbine. Electricity can be sent to resistance heaters immersed in water, or the wind turbine's rotor shaft can be mechanically coupled to a paddle or pump that agitates water, thereby heating it.
Resistance Heaters
The first method of heat storage involves electrical resistance heaters which can be DC or AC powered with unregulated voltage and frequency levels. Thus, the buyer has considerable flexibility in choosing a machine without the need for additional complex and expensive control or conditioning devices. The conversion efficiency of a resistance heater is nearly 100%, and heat loss is minimized if the water storage tank is well-insulated. Resistance heaters can also be used directly to heat air, as with baseboard electric home heaters.
Mechanical Heating
The second method of heating water is by mechanically agitating it, using either a pump or a paddle. The heat is produced by the large frictional losses that are produced by agitation. This method of heating does not require an electrical generator. Instead, the power from the rotating rotor shaft is used directly. Theoretical conversion efficiencies are nearly 100%, but practical considerations can reduce this considerably. As yet, only a few experimental models of this type of wind system have been tested.
Sizing of Off-Grid Systems
Sizing remote systems is substantially different than sizing a wind system for utility interconnection because remote systems must be designed to supply the entire electrical demand. Before one can size the components of a remote system, one must determine the load requirements of the site. This means quantifying the power demand on a daily and seasonal basis. The goal is to compare the amount of energy needed at different times of the day and year to when it is available on average from the wind. After taking into account the wind's intermittence, you can determine the size and type of energy storage or other energy sources needed to meet your total demand.
Battery storage should be sized large enough to handle at least three windless days. Back-up generators are often included in remote electrical systems as a supplemental backup. They help to power large, infrequently used loads and to preserve the life of the batteries by minimizing the number of times they are completely discharged. Also, they run most efficiently at full load. For these reasons, generators are often sized larger than the average expected load of the system so that they can also charge the batteries at the same time, keeping run time and fuel consumption to a minimum. Many wind system dealers are familiar with remote system designs and can assist you in selecting an optimum, cost-effective system.
Installation, Maintenance and System Life
Many dealers will sell and install a complete package, including wind turbine, tower and electrical work. Wind turbines should be installed by qualified, experienced people. Local codes should be checked to determine if contractors must be licensed. The local building inspector may be required to inspect the completed work. For utility interconnected systems, a utility-designated inspector probably will make an inspection.
By their very nature, wind turbines are subject to extreme physical forces. The tips of a wind turbine rotor can reach speeds of up to 300 mph. Hail, dirt and insects contacting the blades at these speeds can cause wear to the blade edges. Bearings that support the rotor or other moving parts are also subject to wear. The lifetime of these bearings depends on the wind conditions and level of maintenance care.
The manufacturer usually specifies what is required for the maintenance of a wind turbine. The entire wind system, including the tower, storage devices and wiring should be inspected at least once a year. Routine maintenance might include changing the transmission oil, greasing the bearings and visually inspecting the condition of the blades, tower and electrical connections. Instead of doing the maintenance work yourself (which may require climbing the tower), you can arrange a maintenance contract with the dealer.
According to manufacturers, the expected lifetime of wind turbines is 20 to 30 years. Although few manufacturers have been around that long, this prediction is not unrealistic. Some electricity-generating wind turbines built more than 50 years ago are still working today. Just like automobiles and washing machines, wind turbines have to be properly and routinely maintained to maximize their life. Do not be surprised if minor repair work is needed soon after initial operation. A reputable company will make such repairs quickly and at no charge.
|
Wind Technology
|
|
|
The pages described below discuss the hardware and maintenance of today's wind turbines, and how they can be incorporated usefully into the home, farm or business. Access the pages via the green links on the left. Wind Energy Systems
This section defines some of the terms used to describe wind energy systems.
Traditionally, wind turbine designs have been dictated by their function. Probably the most familiar wind turbine is the "Dutch windmill," a design developed centuries ago and used in Europe for grinding grain and pumping water. In the United States, the fan-type "water-pumper" can still be seen throughout the countryside, especially at remote watering wells in the western and plains states. Today's modern wind turbines generate electricity using slender aerodynamic blades and tall towers. Because electricity is an indispensable part of life to most people, wind turbines are often interconnected with utility lines, so that power is always available on demand. However, a wind turbine can also be a valuable part of an energy supply system independent from the utility grid.
Wind and Wind Power
This section describes the characteristics of wind flow and explains how to measure and evaluate wind at a prospective wind turbine site.
Like the weather in general, the wind can be unpredictable. It varies from place to place, and from moment to moment. Because it is invisible it is not easily measured without special instruments. Wind velocity is affected by the trees, buildings, hills and valleys around us. Wind is a diffuse energy source that cannot be contained or stored for use elsewhere or at another time. It challenges us to harness it, but it first demands considerable study.
To be economically practical, a wind turbine should experience year-round average wind speeds of at least 12 mph. Sometimes people say, "The wind always blows at my place," or "I live on the highest hill in the county." These observations, while they may be true, are too simplistic. A careful wind survey should be made before buying a wind system. Wind turbine owners who buy without careful consideration are frequently disappointed in their system's performance. At the going price for a complete wind turbine installation, it makes economic sense to first thoroughly check out your wind resource.
Wind Data
This section displays wind data from Iowa cities and towns in tabular and map formats, and includes conversion tables.
This 6-year project is collecting wind data from across the state and developing maps of the average monthly and annual wind speeds across Iowa using a GIS-based computer modeling technique. Detailed wind resource data is available for 2,346 individual locations in the state. Iowa Wind Resource Assessment has been rated as the most comprehensive public wind data collection project in the United States by the National Renewable Energy Laboratory (NREL).
|
What are renewable fuels?
Renewable fuels, or "biofuels" like ethanol and biodiesel, are liquid transportation fuels made from agricultural crops like corn and soybeans. Ethanol is a clean-burning gasoline-type fuel made by fermenting grain or sugar cane into alcohol. Biodiesel is created from oil seeds (like soybeans) and waste materials like restaurant grease. Both ethanol and biodiesel can be used in everyday cars and trucks in low-level blends or in slightly modified vehicles as substitutes for gasoline and diesel.
Why biofuels?
Biofuels are a proven alternative to oil for transportation. Thirty percent of American fuel is already enriched with ethanol (it improves performance and reduces tailpipe emissions), and biodiesel is the fastest growing alternative fuel. The four branches of the U.S. military run their vehicles on 20% biodiesel blends (B20) at installations around the country, and the Navy is brewing its own biodiesel from mess hall cooking oil. Concerns over rising oil prices, national security, greenhouse gas emissions, and other forms of air pollution are also bolstering interest in biofuels.
|
Why Renewables: Solar Power
|
What is solar power?
Solar power is produced by photovoltaic, or "PV", solar panels and other devices that capture the energy in sunlight and convert it to electricity. This electricity can then be fed directly to a consumer, an electric power grid, or a storage device. Typically, solar panels are installed on the roof of a home or business, and use the power generated to meet the owner's energy needs and provide surplus electricity to the grid. Other applications include heating water and providing power in areas where electricity connections are not available, such as on road signs, cellular phone towers, and satellites.
What are the benefits of solar power?
- Competitive Costs: Over the past 20 years, the price of solar power has fallen dramatically and is expected to continue to fall to levels competitive with other sources of electricity. Current systems provide power at approximately $.20 per kilowatt hour and are expected to fall to approximately $.10 per kWh by 2010. These rates are very competitive with average 2005 national retail electricity price of $.08 per kWh and well below many average 2005 regional retail electricity prices such as New England ($.1179 per kWh), the Mid-Atlantic ($.1083 per kWh) and California ($.1131 per kWh). Average residential and commercial electricity rates are even higher, making installing PV systems even more competitive at homes and businesses where solar power would replace electricity purchased from the grid. Additionally, solar power provides consistent, low maintenance electricity and insulates owners from price spikes, blackouts, and market manipulation. Furthermore, excess solar power from a home or business can be sold into local electricity grids, further reducing a building's electricity costs. The sum of these savings over time will pay often surpass a solar power system's installation and maintenance costs - in essence earning money for it's owners.
- Abundant Supply: Solar power could meet today's total U.S. electricity demand by PV systems covering only 0.4% of the nation in a high-sunlight area such as the Southwest — an area about 100 square miles. These panels, in reality, will be installed across the United States on roofs and other structures close where it is consumed. And technologies such as PV roof shingles, windows, and flexible fabrics that are easily and cheaply integrated into new and existing buildings are emerging.
- Secure and Stable Supply: Because solar power is generated domestically, often at the site where it will be consumed, prices and supplies are immune to blackouts, international uncertainty and do not rely on long-distance supply networks.
- Cleaner Air: Solar power does not pollute the air or water. It replaces electricity generated from facilities powered by coal, natural gas and other non-renewable fuels, eliminating threats to public health such as carbon monoxide, particulate, and toxic chemical emissions from those facilities. Additionally, when a solar power replaces electricity from a coal-fired power plant it also eliminates a potential source of sulfur emissions - a major component of acid rain.
- Reducing Global Warming: Solar power does not produce CO2 or any other greenhouse gases, thus helping to reduce the risk of climate change.
Can I install solar panels on my home or business?
Most likely, yes. And, you will likely benefit from government rebates and/or tax incentives that will significantly reduce the installation costs of a solar system. Solar panels are easily integrated into the existing wiring of a home and will work in concert with the electricity grid to power your home or business.
Is solar power reliable?
Solar panels do not have any moving parts and require very little maintenance. They can be installed to augment a building's existing connection to the local electricity grid or, with proper sizing and the addition of batteries or other power storage device, to allow a building to be independent of the grid. In both cases, if demand surpasses the output of the solar panels, for instance overnight or during an unlikely malfunction, they will still provide a reliable supply of electricity. |
Why Renewables: Geothermal Power
r
|
What is geothermal power?
Geothermal power is thermal energy from the earth that is used to heat buildings and generate electricity. Geothermal energy is transported in very hot water and steam via wells or fissures in the earth's crust to its point of use. The water or steam is then distributed through pipes or is used to drive turbines that produce electricity. Because the heat is constantly generated by the natural radioactive decay of rocks at the earth's core, geothermal energy is a renewable and abundant source of energy.
What are the benefits of geothermal power?
- Competitive Price: Wholesale prices for geothermal electricity range from $0.03 to $0.07 per kilowatt hour. At this price, geothermal electricity is competitive with fossil fuel based electricity sources. Additionally, prices have dropped by 25% in the past 2 decades and continue to drop. The goal of the geothermal industry and the the U.S. Department of Energy (DOE) is to bring average geothermal electricity prices to down to $0.03 per kilowatt hour.
- Abundant and Consistent: Currently, installed geothermal electricity facilities produce enough electricity to power approximately 1.6 million U.S. households. U.S. DOE expects prices to continue to drop, bringing an additional 10,000 megawatts of geothermal capacity online within 10 years. This capacity will generate power to meet the needs of nearly 10 million households. Additionally, geothermal power is among the most consistent form of energy available today. Geothermal facilities typically produce power over 90% of the time, compared with 71% for coal fired power plants and fewer than 30% for natural gas fired power plants. And it is immune to international supply disruptions and market manipulation.
- Clean: Extracting the hot water and steam from the earth does not require any fossil fuels and produces little to no nitrous oxide or sulfur-bearing gases. Geothermal electricity replaces electricity generated from facilities powered by coal, natural gas and other non-renewable fuels, eliminating threats to public health such as carbon monoxide, particulate, and toxic chemical emissions from those facilities. Finally, a geothermal power plant takes up very little area and does not require any fuels to be mined or extracted elsewhere, so there are no mine tailings or oil spills to contain or clean up.
- Reduces Global Warming: Geothermal power produces very few emissions of CO2 or other greenhouse gases and can replace sources with high greenhouse gas emissions.
Where can we develop geothermal power?
Good geothermal electricity sites need to have hot geothermal flows at or close to the earth's surface and should be close to transmission lines. In the U.S., most geothermal sites are concentrated in the western states. The first geothermal electricity facility in the U.S. opened in Sonoma Country, California, in 1960 and continues to operate today. Since then, facilities have opened in areas stretching from New Mexico to Washington and in Alaska and Hawaii. However, other geothermal technologies such as heat pumps and direct-use applications such as for heating and cooling buildings or melting snow can be installed almost anywhere. |
Why Renewables: Hydroelectric and Tidal Power
|
What is hydroelectric power?
Hydroelectric power uses energy from the movement of water to generate electricity. Water flows over a turbine and spins blades connected to a generator. Electricity is then fed to the electric grid, an individual consumer or a storage device. The most common type of hydroelectric generating facility is a dam where water is stored until it is needed to produce electricity. Hydroelectric power is considered renewable, though some people have raised concerns over large hydroelectric dams due to their impacts on ecosystems, water quality, and natural river flow.
What is tidal power?
Tidal power is similar to hydroelectric power as it makes use of moving water to spin a turbine to produce electricity. As tides rise and fall due to the gravitational pull of the sun and moon, water flows through the mouths of bays and other narrow points. Tidal power facilities place turbines in these currents or trap water at high tide to release through turbines later. As the tides are generated by the ongoing movements of the planet, tidal power is considered renewable.
What are the benefits of hydroelectric and tidal power?
- Low Lifetime Cost: Generating energy from flowing water is very simple and cheap. While constructing dams and turbines require high initial investments, ongoing costs are very low as the water is typically free.
- Reliable and Predictable: Hydroelectric and tidal power facilities produce power on a predictable, and in the case of hydroelectric dams that store their energy source until it is needed, controllable schedule. They are immune to international energy market fluctuations, and they can rapidly adjust their output to meet demand, often faster than fossil fuel based power plants.
- Cleaner Air: Hydroelectric and tidal power do not produce the air pollution from electricity generation facilities powered by coal, natural gas and other non-renewable fuels. Avoiding carbon monoxide, particulate, and toxic pollution emissions can prevent many tens of thousands of premature deaths in the United States each year from respiratory and heart diseases. When a hydroelectric or tidal power replaces electricity from a coal-fired power plant it also eliminates a potential source of sulfur emissions – a major component of acid rain.
- Climate Mitigation: Hydroelectric and tidal power facilities produce zero emissions of CO2 or other greenhouse gases.
- Flood Protection: Dams can protect downstream communities and businesses from damaging flooding.
- Energy Storage: Dams and tidal barriers can also be used to store energy generated elsewhere by employing pumps to move water against gravity so it may be used later. For instance, electricity generated from a wind farm when there is low electricity demand could pump water from a lower reservoir to a higher reservoir. The energy used to move this water can then be converted back to electricity by releasing that water through the dam's hydroelectric turbines at times of high demand for electricity, in essence timing wind power production to demand.
What is the potential for increasing hydroelectric and tidal power output in the future?
Over the past century, most high quality hydroelectric dam sites have been developed or are protected from development for specific reasons. Today, hydroelectric provides roughly 6.5% of domestic electricity generation. However, there is a growing market for small-scale hydroelectric turbines that can provide limited distributed energy production. In suitable locations, these smaller generators can compete on cost with other distributed generation technologies.
Promising sites for large scale tidal power installation are rare and pose recreational and environmental problems due to the barriers necessary to capture the tides for generation. However, technologies such as turbines that do not rely on tidal barriers are being developed that produce energy from the ongoing currents and tidal flows. These could be more flexible and appropriate to the many variable coastal conditions, but it remains to be seen if and how they fit into the energy market.
Are dams and tidal power installations threats to fish and wildlife?
Diverting water from its natural course does affect an ecosystem, often far upstream and downstream from the dam or tidal power site. However, progress continues to be made to overcome these impacts and ensure that the river systems and tidal basins remain healthy and supportive of the flora and fauna that depend on them. These power systems can not be considered renewable unless they provide for the continued health of their host ecosystems and take action to prevent their long-term problems such as siltation and changes to groundwater supplies. |
Why Renewables: Energy Efficiency
|
What is energy efficiency?
Energy efficiency is simply the ability to do more work with less power. More specifically, it refers to technological improvements that maximize the efficient generation, transmission, storage and/or use of energy. For example, some well known improvements in energy efficiency include compact fluorescent light bulbs, gas-electric hybrid automobiles, and combined heat and power or cogeneration systems. Efficiency can also be viewed more broadly to include approaches such as increased public transportation, distributed electricity generation to reduce transmission losses, and community planning to reduce driving or prevent urban "heat islands" that increase cooling demand.
What are the benefits of energy efficiency?
- Cost Savings and Competitiveness: Efficiency improvements save consumers money and improve business competitiveness. Designing and improving factories, cars, homes and transportation systems to maximize energy efficiency reduces future energy bills and makes businesses more competitive. Repeated studies show that energy efficiency improvements produce high returns on investment and short payback periods when compared with other home and business improvement opportunities.
- Strengthening Local Communities: Energy efficiency improvements are investments in local infrastructure, existing businesses, and American ingenuity. This distributed approach can strengthen American communities by creating local jobs, making existing businesses more competitive, and keeping capital in the local economy. By reducing the demand on existing transportation and energy transmission infrastructure, it frees local governments to invest more in schools, health care, and public safety.
- Reducing Foreign Energy Imports: Improved efficiency lowers demand for energy. Reduced demand has many ancillary benefits beyond lower energy bills, including increased energy independence, insulation from energy price fluctuations, and reduced strain on our military for protecting the energy supply chain.
- Healthier Environment: Reducing demand for energy also reduces the carbon monoxide, particulate, and toxic pollution emissions that come from producing energy. Eliminating the demand for electricity from a coal-fired power plant also eliminates a potential source of sulfur emissions - a major component of acid rain.
- Climate Mitigation: Lowering energy demand reduces the nation's greenhouse gas emissions from fossil fuel combustion.
How can I improve the energy efficiency of my home or business?
There are more ways to improve efficiency than can be suitably discussed here. For home and personal energy use, it can be helpful to analyze your utility bills, research local utility and government efficiency programs and incentives, and explore the many efficiency resources and publications available through your local library or from energy efficiency organizations such as the U.S. Department of Energy's Energy Efficiency and Renewable Energy program and the American Council for an Energy-Efficient Economy. Businesses and industrial facilities can also consult similar resources, or may have more specialized needs and should consult their energy provider, government energy agencies, experienced energy managers, or industrial design experts.
Can energy efficiency meet the energy needs of a growing economy? Won't more production be necessary?
Energy efficiency is an important component of the larger solution to America's energy future. The vast opportunities for efficiency improvements can make the nation more prosperous, more secure, and reduce the strain on our energy infrastructure. Combined with other energy technologies, energy efficiency measures can ensure that America's energy needs are met and its economy will continue to grow and prosper in a sustainable way. |
GEOTHERMAL SYSTEMS
Geopac Eco - 8Kw to 61Kw
LOW AND HIGH TEMPERATURE, REVERSIBLE GROUND SOURCE HEAT PUMP
LCD multi function control panel and time display, ‘Total control’ Siemens computerised programming system. Low noise leval. Easy to install, ready to plug in, full installation and operation details. Alarm system. Automatic safety valve. Outside air temperature sensor. Auto anti freeze system. Single or double Hitachi compressors. Fully adjustable flow output temperature for underfloor heating systems. Suitable for use with all geothermal ground source capture methods (ground loop, vertical, water and slinky) High temperature models ideal for existing boiler replacement. 2 YEAR GUARANTEE
|
ECO - 8KW
Output - 8kw
COP - 4.2 (boW35)
Power supply - 230V/50hz
Refrigerant - R407C
Dimensions - 1000x600x600
Weight - 105kg
AVAILABLE IN HIGH TEMERATURE FORMAT
|
ECO - 10KW
Output - 10kw
COP - 4.3 (boW35)
Power supply - 230V/50hz
Refrigerant - R407C
Dimensions - 1000x600x600
Weight - 110kg
AVAILABLE IN HIGH TEMERATURE FORMAT
|
ECO - 13KW
Output - 13kw
COP - 4.4 (boW35)
Power supply - 440V/50hz
Refrigerant - R407C
Dimensions - 1000x600x600
Weight - 120kg
|
ECO - 15KW
Output - 15.5kw
COP - 4.3 (boW35)
Power supply - 440V/50hz
Refrigerant - R407C
Dimensions - 1000x600x600
Weight - 130kg
|
|
|
|
|
|
|

|
Geopac Eco HP - 20Kw to 31Kw
HIGH PERFORMANCE LOW TEMPERATURE, REVERSIBLE GROUND SOURCE HEAT PUMP
|
ECO HP - 20KW
Output - 20kw
COP - 4.3
Power supply - 440V/50hz
Refrigerant - R407C
2 compressors scroll
Dimensions - 1139x690x1139
Weight - 270kg
|
ECO HP - 25KW
Output - 25kw
COP - 4.4
Power supply - 440V/50hz
Refrigerant - R407C
2 compressors scroll
Dimensions - 1139x690x1139
Weight - 280kg
|
ECO HP - 31KW
Output - 31kw
Power supply - 440V/50hz
Refrigerant - R407C
2 compressors scroll
Dimensions - 1139x690x1139
Weight - 310kg
|
|
|
|
Fuel Cell Systems & Solutions
|
Fuel cell and hydrogen systems for stationary and mobile use are now commercially available for the ever increasing demand of end-users, OEMs and industrial customers wishing to capitalize on the unique capabilities of fuel cell technology for their applications.
Usually the potential user does not have the experience and knowledge in integrating fuel cell and hydrogen supply technology for their application.
We offers a complete range of fuel cell and hydrogen solutions in the following fields:
- Customized laboratory or field demonstrators
- Portable and stationary off-grid power supply solutions
- Electrical vehicle niche applications
Our Systems & Solutions department is ready to serve your needs!
fuel cell technology
how the technology works
How the Ballard® fuel cell works
Download Windows Media Video (12,587k)
Download Quicktime Video (12,017k)
The core of the Ballard® fuel cell consists of a membrane electrode assembly (MEA), which is placed between two flow-field plates.
The MEA consists of two electrodes, the anode and the cathode, which are each coated on one side with a thin catalyst layer and separated by a proton exchange membrane (PEM). The flow-field plates direct hydrogen to the anode and oxygen (from air) to the cathode.
When hydrogen reaches the catalyst layer, it separates into protons (hydrogen ions) and electrons.
The free electrons, produced at the anode, are conducted in the form of a usable electric current through the external circuit. At the cathode, oxygen from the air, electrons from the external circuit and protons combine to form water and heat.
Parts of a Ballard® fuel cell
Expanded Single Fuel Cell
A single fuel cell consists of the membrane electrode assembly and two flow-field plates.
Hydrogen
Hydrogen flows through channels in flow field plates to the anode where the platinum catalyst promotes its separation into protons and electrons. Hydrogen can be supplied to a fuel cell directly or may be obtained from natural gas, methanol or petroleum using a fuel processor, which converts the hydrocarbons into hydrogen and carbon dioxide through a catalytic chemical reaction.
Membrane Electrode Assembly
Each membrane electrode assembly consists of two electrodes (the anode and the cathode) with a very thin layer of catalyst, bonded to either side of a proton exchange membrane.
Air
Air flows through the channels in flow field plates to the cathode. The hydrogen protons that migrate through the proton exchange membrane combine with oxygen in air and electrons returning from the external circuit to form pure water and heat. The air stream also removes the water created as a by-product of the electrochemical process.
Flow Field Plates
Gases (hydrogen and air) are supplied to the electrodes of the membrane electrode assembly through channels formed in flow field plates.
Fuel Cell Stack
To obtain the desired amount of electrical power, individual fuel cells are combined to form a fuel cell stack. Increasing the number of cells in a stack increases the voltage, while increasing the surface area of the cells increases the current.
Types of Fuel Cells
Alkaline fuel cells - AFC
Alkaline fuel cells were first used in the Gemini-Apollo space program to produce drinking water and electrical energy.
Operate on compressed hydrogen.
Alkaline fuel cells generally use a solution of potassium hydroxide (chemically, KOH) in water as their electrolyte.
Output of alkaline fuel cell ranges from 300 watts (W) to 5 kilowatts (kW).
Direct methanol fuel cells - DMFC
Direct methanol fuel cells use methanol instead of hydrogen.
Operating temperatures of direct methanol fuel cells are in the same range as PEM fuel cells - 50 to 100°C (122 to 212°F).
Direct methanol fuel cells are being considered for use in the transportation industry.
Molten carbonate fuel cells - MCFC
Molten carbonate fuel cells use a liquid solution of lithium, sodium, and/or potassium carbonates soaked in a matrix.
Units with output up to 2 megawatts (MW) have been constructed, and designs exist for units up to 100 MW.
The nickel electrode-catalysts of molten carbonate fuel cells are inexpensive compared to those used in other cells, but the high temperature also limits the materials and safe uses of MCFCs.
Phosphoric acid fuel cells - PAFC
Phosphoric acid fuel cells use phosphoric acid as the electrolyte to make electricity.
Efficiency ranges from 40 to 80 percent and operating temperature is 150 to 200° C (about 300 to 400° F).
Existing phosphoric acid cells have outputs up to 200 kW, and 11 MW units have been tested.
Proton exchange membrane fuel cells - PEM
PEM fuel cells are the most common type of fuel cell being developed for transportation use.
They operate at the one kW per liter of volumetric powered level at a temperature under 100°C (212 °F)
PEM fuel cells react quickly to changes in electrical demand and will not leak or corrode.
PEM fuel cells use inexpensive manufacturing materials (plastic membrane).
Regenerative fuel cells - RFC
Regenerative fuel cells separate water into hydrogen and oxygen by a solar-powered electrolyser.
Hydrogen and oxygen are fed into regenerative fuel cells, generating electricity, heat and water.
Water is then recirculated back to the electrolyser of the regenerative fuel cell and the process repeats.
Solid oxide fuel cells - SOFC
Solid oxide fuel cells use a hard, ceramic compound of metal (like calcium or zirconium) oxides (chemically, O2) as electrolyte.
Output for solid oxide fuel cells is up to 100 kW.
Reformer is not required to extract hydrogen from the fuel due to high temperature.
June 18, 2007
First Solar-Hydrogen Fuel Cell Residence to be Built on Grand Cayman
Press Release from Renewable Energy International
June 19, 2007 - New Jersey, USA:
Renewable Energy International, Inc. (REI), a leading integrator of renewable energy technologies, announced today that it will design and install a groundbreaking renewable solar-hydrogen fuel cell energy system in a residential environment in the Cayman Islands – only the second such system in North America. The U.S. based company holds the distinction of developing the first prototype solar-hydrogen system near Princeton, New Jersey, USA, which was dedicated in October 2006 before international press coverage. This second installation in the Caymans will demonstrate the system's significant cost and environmental benefits in an island environment.
This unique renewable energy system provides complete energy production, indefinite storage, and delivery with no emissions or carbon footprint whatsoever. Unlike most hydrogen fuel cell systems, this one is powered only by solar energy, making it the cleanest hydrogen generation system in the world.
“The installation of this solar-hydrogen system on the Grand Cayman will be the first carbon-neutral building in the Caymans.” noted Michael Strizki, inventor of the REI solar-hydrogen system. “We will design a solar-hydrogen system for a 3,000 sq. ft. private residence on Grand Cayman Island. Design stage commenced on June 4th and installation will follow”, said Strizki. “This solar-hydrogen power system will demonstrate that the reality of a completely renewable energy system is no longer in the future, it is here now. We have the ability to replicate this system for remote homes, commercial buildings, for backup storage for essential community buildings such as hospitals…and eventually entire communities. We hope to set an example here for all island communities to follow”.
REI’s design for this installation will closely replicate the prototype system in New Jersey. It will include a photovoltaic array that will produce electricity to provide for the home’s primary electricity load, as well as enough excess electricity to run an electrolyzer which converts water into hydrogen. The hydrogen produced will be reserved in a storage tank until needed, at which point it will be converted back into electricity through means of a fuel cell. To increase energy efficiency, the system will be integrated with a geothermal system that will provide for the home’s total cooling needs, as well as its domestic hot water. Energy efficient appliances will also be installed at the home to reduce the overall electricity load. Heat, although available, will not be required due to the climate in the Cayman Islands.
The solar-hydrogen system will be designed to meet the home’s total energy needs without reliance on any external source of energy. However, the home will remain grid-connected at the request of the homeowner, and with the support and approval of the Caribbean Utility Company (CUC).
This installation is expected to be significantly more cost effective and energy efficient than the first solar-hydrogen system designed by REI, primarily due to the fact that it will be installed in a sunny island environment. As the Caymans receive an average of 345 days of sunlight annually, the system will require a much smaller solar array, as well as reduced hydrogen production and storage capability, bringing down system costs significantly. The system is expected to be completed for less than US $200,000.00, representing a cost reduction of 60% over the prototype system. Mr. Jim Knapp, owner of the residence on Grand Cayman where the system will be installed, pointed out that “The environmental benefits are important, but not the only consideration. For me, this is a financial play. The numbers work right now to make this a smart move.”
Mr. Gian-Paolo Caminiti, COO of REI, explained the further significance behind the project: “The island environment is a perfect application of this technology; it is a remote location requiring imported fuel to generate power, has abundant solar power available, and no current systems in place to harness the energy. Installing a distributed (not connected to a central generation station) system here to generate power from a sustainable, carbon-neutral source makes perfect sense. One system does it all; total energy needs are met all year long and, in the event of a hurricane or other disaster, backup power without any supply line dependency or interruption is secured. The potential applications for this throughout the region, and the world, are enormous.”
Electricity production in island environments is both carbon-intensive and extremely expensive, at roughly three times the cost of electricity in the United States. Currently, the Cayman Islands import 100% of their electricity, which is produced by fossil-fuel based generation methods, consuming over 400 million kilowatt hours (kWh) annually. As all of the electricity consumed is generated off-island and imported, additional transportation costs and adverse environmental impacts are incurred, including increased carbon dioxide emissions.
The Cayman Islands are an overseas territory of the United Kingdom, whose government is now considering sweeping legislation to mandate sharp cuts in carbon emissions. Ms. Gina Ebanks-Petrie, Director of the Cayman Islands Department of the Environment commented favorably on the project. “Given the challenges posed by climate change for our islands, the Cayman Islands Department of Environment very much supports the use of renewable sources of energy. We are extremely pleased to see private individuals taking the initiative in this area. The Cayman Islands Government has recently signed the United Nations Framework Convention on Climate Change and its Kyoto Protocol, so a national discussion needs to take place in the very near future on, among other things, the appropriate mix between renewable and traditional energy sources in meeting the country’s demand for electricity. ”
For Further Information
We are solar powered
Many energy options in the WilderHill Index (ECO) are technologies we not only address in an abstract sense, but also use in applied settings everyday. We believe that the practical knowledge gained this way, can assist when discussing clean energy ahead. For example, we're intimately familiar with the diverse ways solar power may be sensible today. This is more than theoretical; at our 1-acre San Diego site, we utilize solar as a means to:
- generate the electricity for our home/office demonstration site;
- provide thermal hot water for the building;
- heat a 40,000 gallon swimming pool;
- provide night lighting; and
- run an electric bike.
For example, we're harvesting considerable electric power from the sun, via solar PV (PhotoVoltaic = electricity generating) panels. This array is 'grid-intertied', meaning that our building is connected to the power-grid; during the daylight hours, we're generally making more power than we consume, and so we automatically 'sell' excess power back into the grid. Thus in daylight, our electric meter actually runs backwards. After sunset, it's reversed; we then 'buy' our power from the utility - and the meter runs the opposite way. We seek to make enough power during daylight, to make up for night, and to have a zero utility bill. Grid-intertied means we're connected, able to avoid the cost of batteries, and it permits rebates from the State of California. As shown next, for cost breakdown, the system performs well in cost/benefit projections, return on investment, and in practice.
This 3.85 kilowatt (kW) solar PV system is calculated to achieve payback, in approximately 7 - 10 years (see solar PV system costs). That reasonable time to payback, is due *to California State solar PV subsidies cutting the PV system's original costs in half, *to coming time-of-use (TOU) metering by our utility that allows us to 'sell' electricity at an attractive rate, and *to a California tax credit. Certainly, the upfront costs for the whole PV system were significant. These costs were $15,000, but California subsidies and tax credit meant that we only paid about half of what the system would cost, without those benefits.
We've estimated that after generating solar power for 7 - 10 years, we'll recoup full return on investment, and so thereafter most of our electricity is made at no charge. That stability contrasts with dynamic local utility rates that usually go up over time. Smartly, our fixed cost and equipment will go on helping us, for many years, after cost amortization. Solar panels are the main cost here, and they carry a manufacturer's Warranty of 25 years - notably, a longer life should be expected from these panels, given the performance seen from panels in use over decades. The 3.85 kW Inverter carries a shorter life of perhaps 7 - 10 years and will have to be replaced. We expect years of profitable PV operation and thus feel without any undue sentiment, that this can be a sensible return on investment.
This system has performed without a hitch. In 2003, we installed the 21 then-new Sharp 185 watt panels (see spec sheet) in three strings with each panel at a 14.2% efficiency rating. These panels were made in the USA, and were then among the most efficient of any PV consumer product, employing a rather unique and power-dense mono-crystalline design. These panels were matched to a Sharp Sunvista 3,500 watt inverter (along with two web-based real-time monitoring systems) in one of the first such installations in California.
As illustrated by daily monitoring data in detailed graphs, we've been obtaining module efficiencies of roughly 5% to 10% over the manufacturer's rating. Inverter efficiencies are also measurably high, and in Spring through Summer months we can generate over 20 kilowatt hours per day. During Fall or Winter months, with fewer daylight hours, the sun much lower, and less irradiation overall, we may generate well less than half that amount.
 |

Solar PV panels being installed, 2003 |
Besides the PV that's used to make our electric power, we also use two independent solar water-heating systems. Both are thermal, and so they rely instead on simply collecting the sun's warmth to heat water. First there's one system that's completely passive and doesn't require pumps, thus increasing overall efficiency; this also extends expected system life. It provides daily hot water for our home/office, in a roughly 2,500 square foot building.
The second thermal system, is used for heating a large pool. Currently the system relies on an existing one horsepower pump already filtering the pool, since that pump can on sunny days circulate water through the panels too. The pump is powered by the home PV array + inverter, to move water through 400 square feet of thermal panels (10 panels that are 10 foot by 4 foot each), heating the pool significantly Spring to early Fall. Throughout the summer, the pool generally stays around 87 degrees F due to this solar thermal heating. But that AC pump, which is on for many hours per day, is a large consumer of home PV power.
|

Smaller, passive thermal water heating |
|

Large panels for heating pumped-water. |
We closely monitor our building energy demand - as well as solar power generated - because it's as useful to reduce our demand, and so avoid a need for power in the first place, as it is to make energy renewably. Energy demand is thus displayed live above. While these surprisingly simple efficiency steps that we'll advocate for, may be given less public attention than innovative ways to make power, we believe there's ample reasons to act first to reduce the need for power. Many solutions we've adopted are indeed compelling for their very simplicity, and widely repeatable. Among these easy, reproducible steps are:
- We use compact fluorescent lights, instead of hot, incandescent bulbs: this also reduces a need for air conditioning in summer, exacerbated by bulbs in the first place;
- We use a simple retractable awning to shade the windows on the South of the building. On hot days, we flip a switch extending the awning for shade when the sun's high; on cool days we leave the awning retracted to let sunlight heat much of the building. This system could conceivably be automated. We've also planted deciduous trees on the building's South-side, to add shade in summer, while bare branches let sun through in Winter;
- We seek energy-efficient appliances in the first place. By thoughtful shopping, we found a 55 inch HD TV diode projection TV using 280 watts, roughly half that of a plasma TV. Yet ours has arguably better picture quality, than plasmas consuming far more energy;
- We use small 1-watt white led emitting diodes (WLEDs) together with solar PV;
- One large lighting structure here could traditionally use 12 (hot) bulbs for 720 watts: by using more efficient lights, we consume about 75% less power for appropriate lumens - and again, without added heat;
Additions Sought Next:
- We now have an electric bike, that's charged using solar power. However, a full-sized electric vehicle (EV) powered by solar would be much compelling in this home/office site. We seek to bring in an electric car, to demonstrate ways to move off carbon and gasoline, and to use solar/wind to run both the building, and vehicle in everyday chores;
- We also seek to bring in a fuel cell. For example a 5 KW FC that supplements our combined solar PV, wind, and micro-hydro power could together run the building, plus easily fuel an electric car too. This fuel cell would best run off 'green hydrogen' that's made by surplus PV/wind and electrolysis: or, it could run off a more conventional fuel such as propane;
- We seek to bring in a small wind turbine, 0.5 to 2 kilowatts in size. We do have some limited but usable wind resources on this 1-acre demonstration site;
- We seek to bring in micro-hydro power too, since our site has a seasonal stream running through it with a sizable head & drop above. This could be a small demonstration unit;
- We seek to bring in another 600 watts of solar PV panels, to run a dedicated DC pool pump directly off the sun. This can demonstrate efficient pumping that also eliminates building load, facilitates solar thermal, and renders the building entirely solar-powered to boot.
We welcome your thoughts and suggestions. Notably, requests over five years regarding our energy and investing ideas, helped us to conceive the WilderHill Clean Energy Index (ECO), the first Index on Wall Street for renewable energy solutions - now successfully run by our 'sister' for-profit group, WilderShares, LLC. After much encouragement, we've recently grown here too at the Hydrogen Fuel Cell Institute, and have become a 501c3 organization now able to receive tax-deductible donations to support our vision for a clean energy tomorrow. As we build from a modest showcase of energy solutions, we're working towards solar/wind-renewable powered buildings, cars, & fuel cells based on 'green hydrogen'.
Project Description
IPRO Project “Solar Sign”:
The “Solar Sign” IPRO started in the Spring semester of 1997. In eight semesters, over 75 graduate and undergraduate IIT students from the Chemical, Environmental, Civil/Architecture, Mechanical, Electrical, and Computer Engineering have participated in this project. The objectives of the project were to design, assemble and demonstrate a stand-alone distributed power generation system which utilizes solar energy through PV panels to power a light emitting diode (LED) sign. The purpose of the sign is to promote solar energy and other clean energy technologies by projecting daily messages. The sign is visible to commuters on heavily traveled Chicago highway and visitors to Comiskey Park, home of the Chicago White Sox. The project has been conducted in two phases. In Phase I, the energy generated from the sun was stored in a large nickel-cadmium (Ni-Cd) battery bank. In Phase II, a solar hydrogen system (proton exchange membrane (PEM) electrolyzer, PEM fuel cell, and H2 storage unit) is being installed.
Solar powered photovoltaic (PV) systems have numerous applications and can be designed to provide energy demands from a few watts to kilo- or megawatts [3-4]. Some typical PV applications are: communication, monitoring and sensing, water pumping, corrosion protection, lighting, and refrigeration, to name a few. Solar energy is seasonal, varied and intermittent. Therefore, energy storage, conversion and delivery to satisfy application loads are key system design criteria for specific PV applications.

Figure 2: Statistics for year 1995-2000 and future plans for year 2001-2003 for the number of IPRO projects at IIT.

Figure 1: Schematic diagram of the IPRO structure at IIT
Solar Panels
The solar panels were received as a matchable gift from Solarex Corporation. A total of 2.2 kWp of the panels are used in the PV set-up, of which 1.2 kWp are multi-crystalline silicon and the remaining are amorphous silicon. Their efficiencies are within 10-15%. A pyranometer measures the daily insolation and shunt resistances within the array current. Over a given time the array voltage and current are acquired as a function of insolation. The array current integrated over a period of time gives the amount of charge that is available for charging the battery packs. At present both arrays are being used to charge the batteries.

Figure 4: Performance results for the multi-crystaline and amorphous silicon PV panels powering Phase I of the system.
Power in: measured solar insolation
Power out: electricity generated by the PV panels
Efficiency = Power in
Power out

Figure 5: Breakdown of the results shown in Figure 4, for overall system charge and cumulative electric generation/consumption of Phase I system during a four-day continuos test.
Inverter / DC-DC Converter
In a fully automated system, the combination of inverter charge controller serves to terminate either charge or discharge while making sure that the batteries are neither overcharged nor over-discharged. At the same time, the inverter serves to insulate the battery power supply from the PV panels to engage the grid, if needed, to deliver energy to a load such as the illuminated IIT sign. Proper integration of the inverter funct ion with the balance of the system, particularly the battery sub-system, is essential. Otherwise, the inverter may act as a battery load, thereby causing the battery to discharge.
Batteries
In spite of many shortcomings, batteries are still a preferred way of storing electrical energy generated by the PV cells. Electrical energy is changed to chemical energy in batteries when they are charged from solar panels during the daytime and electrical power is delivered to the load when the batteries are discharged during the nighttime to power the LED sign.
Overall battery size, which depends upon the system load demand, available solar power, and system autonomy requirements, is an important element in the design, operation and maintenance of PV/battery systems.
Individual battery parameters, including voltage, capacity and cycling characteristics, are also quite important for battery sizing, design installation and operation. In the preliminary IPRO project, nickel/cadmium batteries manufactured by Alcad were received (2/3 of the batteries were donated while IIT covered 1/3 of the cost). The system contains thirty-eight nickel/cadmium batteries having a nominal voltage of 1.2V on open-circuit and a capacity of 300 Ah. The total available energy from the batteries to the loads is estimated at 13.68 kWh or 2.7 days (of 10 night hours per 24h) to operate a 500 W load.
In the nickel/cadmium batteries, nickel oxy-hydroxide is the cathode and cadmium is anode. In spite of the toxic element cadmium and the cost of nickel, nickel/cadmium batteries were selected in the preliminary IPRO project because they are suited for low temperature applications (the electrolyte does not freeze even at –200C) and the battery life is approximately 5 times longer than that of a lead-acid battery. The life expectancy of a nickel/cadmium battery, when properly handled without
overcharging or over- discharging) may be up to 1500 cycles.

Figure 3: Schematics for Phase I of the system for the “Solar Sign” IPRO at IIT.
Electrolyzer
In the proposed project, the electrical energy generated by the PV cells will be stored as hydrogen energy. Many techniques can be used to generate hydrogen. Hydrocarbon conversion, ammonia dissociation, methanol reforming and sponge-iron oxidation techniques generate carbon monoxide (poisonous) and carbon dioxide (polluting) as well as hydrogen. However, water electrolysis produces only hydrogen and oxygen; this is the reason for using an electrolyzer to provide hydrogen to the fuel cell.
Theoretically, electrolyzers split water into hydrogen and oxygen at an electrical potential of 1.23 volts. In reality, the voltage may be 30-40% higher. There are two main types of electrolyzers, named after the electrolyte used: the potassium hydroxide (KOH) electrolyzer, and the Proton Exchange Membrane (PEM) electrolyzer. In either type, electrocatalysts must be used to split the water molecules at 1.60-1.70 V. An advantage of PEM electrolysis is that it can produce pure hydrogen gas
at pressures around 200 psi or higher. This eliminates the need for compressors in storing the gas.

Figure 6: Block diagram schematic for Phase II of the system for the “Solar Sign” IPRO at IIT.

Figure 7: Polarization test results for the 200 W PEM fuel cell (200 W Power PackTM, Electrochem Corp., Boston, MA) used in Phase II of the System.
Hydrogen Storage
Hydrogen is now recognized to be the optimal energy carrier for sustainable energy systems, and is expected to become the transportation fuel of choice, sometime during this century. Hydrogen may be stored in gaseous, liquid, or solid form (the latter mainly as metal hydride or in carbon). The type of application mainly determines the method of storage, for example liquid hydrogen nowadays is primarily used as a rocket fuel because it has the lowest boiling point and density, and the highest specific thrust of any known fuel. Models presented in the literature describe each type of hydrogen storage and explain its advantages and disadvantages in terms of cost, stability, convenience of usage, and energy density.
Although it is advisable to store hydrogen in the gaseous or liquid state, several problems like energy consumed in compression or liquefaction and boil-off losses during storage, stand in the way of large-scale and long-term storage. These problems will be addressed in this project, to recommend an optimum method to store hydrogen for power generation.
In this project and for practical considerations, we will focus on hydrogen storage in a compressed-cylinder using a small-scale compressor. We will also look into other means of storage using light-weight hydrogen storage
cylinders.
Fuel Cells
Fuel cells will be used to deliver the stored chemical energy of hydrogen.Fuel cells are electrochemical devices capable of delivering electrical energy continuously through reaction of a fuel and an oxidant gas, at efficiencies within 50-60% range. There are many different types of fuel cells, distinguished by their electrolyte and operating temperature. Only the
relatively low-temperature fuel cells are attractive for the PV/electrolyzer/fuel-cell/hydrogen-storage application. Therefore, the
PEM fuel cell, which operates at approximately 80 oC is the best choice, although its cost is still very high (i.e., the 200 W PEM fuel, cell used in this project will cost $7,500).
|
|
|
|
|
|
|
|